Abstract
Comprising ~20 % of the volume, water is a key determinant of the mechanical behavior of cortical bone. It essentially exists in two general compartments: within pores and bound to the matrix. The amount of pore water—residing in the vascular-lacunar-canalicular space—primarily reflects intracortical porosity (i.e., open spaces within the matrix largely due to Haversian canals and resorption sites) and as such is inversely proportional to most mechanical properties of bone. Movement of water according to pressure gradients generated during dynamic loading likely confers hydraulic stiffening to the bone as well. Nonetheless, bound water is a primary contributor to the mechanical behavior of bone in that it is responsible for giving collagen the ability to confer ductility or plasticity to bone (i.e., allows deformation to continue once permanent damage begins to form in the matrix) and decreases with age along with fracture resistance. Thus, dehydration by air-drying or by solvents with less hydrogen bonding capacity causes bone to become brittle, but interestingly, it also increases stiffness and strength across the hierarchical levels of organization. Despite the importance of matrix hydration to fracture resistance, little is known about why bound water decreases with age in hydrated human bone. Using 1H nuclear magnetic resonance (NMR), both bound and pore water concentrations in bone can be measured ex vivo because the proton relaxation times differ between the two water compartments, giving rise to two distinct signals. There are also emerging techniques to measure bound and pore water in vivo with magnetic resonance imaging (MRI). The NMR/MRI-derived bound water concentration is positively correlated with both the strength and toughness of hydrated bone and may become a useful clinical marker of fracture risk.







Similar content being viewed by others
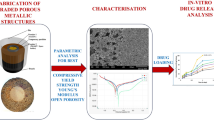
References
Currey JD (1989) Biomechanics of mineralized skeletons. In: Carter JG (ed) Skeletal biomineralization: patterns, processes and evolutionary trends. American Geophysical Union, Washington, D.C., pp 11–25
Mueller KH, Trias A, Ray RD (1996) Bone density and composition. Age-related and pathological changes in water and mineral content. J Bone Joint Surg Am 48:140–148
Robinson RA (1979) Bone tissue: composition and function. Johns Hopkins Med J 145:10–24
Dong P, Haupert S, Hesse B, Langer M, Gouttenoire PJ, Bousson V, Peyrin F (2014) 3D osteocyte lacunar morphometric properties and distributions in human femoral cortical bone using synchrotron radiation micro-CT images. Bone 60:172–185
Robinson RA (1975) Physicochemical structure of bone. Clin Orthop Relat Res 112:263–315
Wang Y, Von Euw S, Fernandes FM, Cassaignon S, Selmane M, Laurent G, Pehau-Arnaudet G, Coelho C, Bonhomme-Coury L, Giraud-Guille MM, Babonneau F, Azais T, Nassif N (2013) Water-mediated structuring of bone apatite. Nat Mater 12:1144–1153
Marino AA, Becker RO, Bachman CH (1967) Dielectric determination of bound water of bone. Phys Med Biol 12:367–378
Maeda H, Fukada E (1982) Effect of water on piezoelectric, dielectric, and elastic properties of bone. Biopolymers 21:2055–2068
Robinson W (1931) Free and bound water determinations by the heat of fusion of ice method. J Biol Chem 92:699–709
Nomura S, Hiltner A, Lando JB, Baer E (1977) Interaction of water with native collagen. Biopolymers 16:231–246
Pineri MH, Escoubes M, Roche G (1978) Water-collagen interactions: calorimetric and mechanical experiments. Biopolymers 17:2799–2815
Armeniades CD, Kuriyama I, Roe JM, Baer E (1967) Mechanical behavior of poly(ethyleoe terephthalate) at cryogenic temperatures. J Macromol Sci Part B 1:777–791
Fraser RD, MacRae TP, Suzuki E (1979) Chain conformation in the collagen molecule. J Mol Biol 129:463–481
Okuyama K, Okuyama K, Arnott S, Takayanagi M, Kakudo M (1981) Crystal and molecular structure of a collagen-like polypeptide (Pro-Pro-Gly)10. J Mol Biol 152:427–443
Lazarev YA, Grishkovsky BA, Khromova TB, Lazareva AV, Grechishko VS (1992) Bound water in the collagen-like triple-helical structure. Biopolymers 32:189–195
Brodsky B, Persikov AV (2005) Molecular structure of the collagen triple helix. Adv Protein Chem 70:301–339
Bella J, Brodsky B, Berman HM (1995) Hydration structure of a collagen peptide. Structure 3:893–906
Lees S (1981) A mixed packing model for bone collagen. Calcif Tissue Int 33:591–602
Wilson EE, Awonusi A, Morris MD, Kohn DH, Tecklenburg MM, Beck LW (2005) Highly ordered interstitial water observed in bone by nuclear magnetic resonance. J Bone Miner Res 20:625–634
Timmins PA, Wall JC (1977) Bone water. Calcif Tissue Res 23:1–5
Mkukuma LD, Skakle JM, Gibson IR, Imrie CT, Aspden RM, Hukins DW (2004) Effect of the proportion of organic material in bone on thermal decomposition of bone mineral: an investigation of a variety of bones from different species using thermogravimetric analysis coupled to mass spectrometry, high-temperature X-ray diffraction, and Fourier transform infrared spectroscopy. Calcif Tissue Int 75:321–328
Mkukuma LD, Imrie CT, Skakle JM, Hukins DW, Aspden RM (2005) Thermal stability and structure of cancellous bone mineral from the femoral head of patients with osteoarthritis or osteoporosis. Ann Rheum Dis 64:222–225
Neuman WF, Neuman MW (1953) The nature of the mineral phase of bone. Chem Rev 53:1–45
Casciani FS (1971) Identification of hydrate water in enamel, dentine, cementum and bone. In: Fearnhead RW, Stack MV (eds) Tooth Enamel II: its composition, properties and fundamental structure. John Wright & Sons Ltd, Bristol, pp 14–23
Cho G, Wu Y, Ackerman JL (2003) Detection of hydroxyl ions in bone mineral by solid-state NMR spectroscopy. Science 300:1123–1127
Rai RK, Sinha N (2011) Dehydration-induced structural changes in the collagen-hydroxyapatite interface in bone by high-resolution solid-state NMR spectroscopy. J Phys Chem C 115:14219–14227
Yoder C, Pasteris J, Worcester K, Schermerhorn D, Sternlieb M, Goldenberg J, Wilt Z (2012) Dehydration and rehydration of carbonated fluor- and hydroxylapatite. Minerals 2:100–117
Davies E, Muller KH, Wong WC, Pickard CJ, Reid DG, Skepper JN, Duer MJ (2014) Citrate bridges between mineral platelets in bone. Proc Natl Acad Sci USA 111:E1354–E1363
Faingold A, Cohen SR, Shahar R, Weiner S, Rapoport L, Wagner HD (2014) The effect of hydration on mechanical anisotropy, topography and fibril organization of the osteonal lamellae. J Biomech 47:367–372
Wenger MP, Bozec L, Horton MA, Mesquida P (2007) Mechanical properties of collagen fibrils. Biophys J 93:1255–1263
Gautieri A, Vesentini S, Redaelli A, Buehler MJ (2011) Hierarchical structure and nanomechanics of collagen microfibrils from the atomistic scale up. Nano Lett 11:757–766
Wess TJ, Orgel JP (2000) Changes in collagen structure: drying, dehydrothermal treatment and relation to long term deterioration. Thermochim Acta 365:119–128
Feng L, Chittenden M, Schirer J, Dickinson M, Jasiuk I (2012) Mechanical properties of porcine femoral cortical bone measured by nanoindentation. J Biomech 45:1775–1782
Guidoni G, Swain M, Jager I (2010) Nanoindentation of wet and dry compact bone: Influence of environment and indenter tip geometry on the indentation modulus. Philos Mag 90:553–565
Hengsberger S, Kulik A, Zysset P (2002) Nanoindentation discriminates the elastic properties of individual human bone lamellae under dry and physiological conditions. Bone 30:178–184
Rodriguez-Florez N, Oyen ML, Shefelbine SJ (2013) Insight into differences in nanoindentation properties of bone. J Mech Behav Biomed Mater 18:90–99
Rho JY, Pharr GM (1999) Effects of drying on the mechanical properties of bovine femur measured by nanoindentation. J Mater Sci Mater Med 10:485–488
Lee KL, Baldassarri M, Gupta N, Pinisetty D, Janal MN, Tovar N, Coelho PG (2012) Nanomechanical characterization of canine femur bone for strain rate sensitivity in the quasistatic range under dry versus wet conditions. Int J Biomater 2012:415230
Seto J, Gupta HS, Zaslansky P, Wagner HD, Fratzl P (2008) Tough lessons from bone: extreme mechanical anisotropy at the mesoscale. Adv Funct Mater 18:1905–1911
Spiesz EM, Roschger P, Zysset PK (2012) Elastic anisotropy of uniaxial mineralized collagen fibers measured using two-directional indentation. Effects of hydration state and indentation depth. J Mech Behav Biomed Mater 12:20–28
Wolfram U, Wilke HJ, Zysset PK (2010) Rehydration of vertebral trabecular bone: influences on its anisotropy, its stiffness and the indentation work with a view to age, gender and vertebral level. Bone 46:348–354
Pathak S, Swadener JG, Kalidindi SR, Courtland HW, Jepsen KJ, Goldman HM (2011) Measuring the dynamic mechanical response of hydrated mouse bone by nanoindentation. J Mech Behav Biomed Mater 4:34–43
Nicolella DP, Ni Q, Chan KS (2011) Non-destructive characterization of microdamage in cortical bone using low field pulsed NMR. J Mech Behav Biomed Mater 4:383–391
Evans FG, Lebow M (1951) Regional differences in some of the physical properties of the human femur. J Appl Physiol 3:563–572
Yin L, Venkatesan S, Webb D, Kalyanasundaram S, Qin QH (2012) 2D and 3D mapping of microindentations in hydrated and dehydrated cortical bones using confocal laser scanning microscopy. J Mater Sci 47:4432–4438
Dempster WT, Richard TL (1952) Compact bone as a non-isotropic material. Am J Anat 91:331–362
Smith JW, Walmsley R (1959) Factors affecting the elasticity of bone. J Anat 93:503–523
Nyman JS, Roy A, Shen X, Acuna RL, Tyler JH, Wang X (2006) The influence of water removal on the strength and toughness of cortical bone. J Biomech 39:931–938
Broz JJ, Simske SJ, Greenberg AR, Luttges MW (1993) Effects of rehydration state on the flexural properties of whole mouse long bones. J Biomech Eng 115:447–449
Nyman JS, Gorochow LE, Adam Horch R, Uppuganti S, Zein-Sabatto A, Manhard MK, Does MD (2013) Partial removal of pore and loosely bound water by low-energy drying decreases cortical bone toughness in young and old donors. J Mech Behav Biomed Mater 22:136–145
Yan J, Daga A, Kumar R, Mecholsky JJ (2008) Fracture toughness and work of fracture of hydrated, dehydrated, and ashed bovine bone. J Biomech 41:1929–1936
Melvin JW, Evans FG (1973) Crack propagation in bone. In: Biomechanics Symposium ASME, New York, pp. 87–88
Kikugawa H, Yasui Y, Tomatsu T (2000) Effect of strain rate on the fracture toughness of cortical bone. J Soc Mater Sci Jpn 49:327–333
Kahler B, Swain MV, Moule A (2003) Fracture-toughening mechanisms responsible for differences in work to fracture of hydrated and dehydrated dentine. J Biomech 36:229–237
Wegst UG, Bai H, Saiz E, Tomsia AP, Ritchie RO (2015) Bioinspired structural materials. Nat Mater 14:23–36
Yamashita J, Li X, Furman BR, Rawls HR, Wang X, Agrawal CM (2002) Collagen and bone viscoelasticity: a dynamic mechanical analysis. J Biomed Mater Res 63:31–36
Garner E, Lakes R, Lee T, Swan C, Brand R (2000) Viscoelastic dissipation in compact bone: implications for stress-induced fluid flow in bone. J Biomech Eng 122:166–172
Sasaki N, Enyo A (1995) Viscoelastic properties of bone as a function of water content. J Biomech 28:809–815
Liebschner MA, Keller TS (2005) Hydraulic strengthening affects the stiffness and strength of cortical bone. Ann Biomed Eng 33:26–38
Eberhardsteiner L, Hellmich C, Scheiner S (2014) Layered water in crystal interfaces as source for bone viscoelasticity: arguments from a multiscale approach. Comput Methods Biomech Biomed Eng 17:48–63
Nalla RK, Kinney JH, Tomsia AP, Ritchie RO (2006) Role of alcohol in the fracture resistance of teeth. J Dent Res 85:1022–1026
Maciel KT, Carvalho RM, Ringle RD, Preston CD, Russell CM, Pashley DH (1996) The effects of acetone, ethanol, HEMA, and air on the stiffness of human decalcified dentin matrix. J Dent Res 75:1851–1858
Smith NW, Ekwaro-Osire S, Khandaker M, Hashemi J (2010) Influence of storage duration on retention of original fracture toughness. Exp Mech 51:697–705
Lucksanasombool P, Higgs WA, Higgs RJ, Swain MV (2001) Fracture toughness of bovine bone: influence of orientation and storage media. Biomaterials 22:3127–3132
Nalla RK, Balooch M, Ager JW 3rd, Kruzic JJ, Kinney JH, Ritchie RO (2005) Effects of polar solvents on the fracture resistance of dentin: role of water hydration. Acta Biomater 1:31–43
Bembey AK, Oyen ML, Bushby AJ, Boyde A (2006) Viscoelastic properties of bone as a function of hydration state determined by nanoindentation. Philos Mag 86:5691–5703
Bushby AJ, Ferguson VL, Boyde A (2004) Nanoindentation of bone: comparison of specimens tested in liquid and embedded in polymethylmethacrylate. J Mater Res 19:249–259
Dall’Ara E, Ohman C, Baleani M, Viceconti M (2007) The effect of tissue condition and applied load on Vickers hardness of human trabecular bone. J Biomech 40:3267–3270
Pashley DH, Agee KA, Nakajima M, Tay FR, Carvalho RM, Terada RS, Harmon FJ, Lee WK, Rueggeberg FA (2001) Solvent-induced dimensional changes in EDTA-demineralized dentin matrix. J Biomed Mater Res 56:273–281
Streeter I, de Leeuw NH (2011) A molecular dynamics study of the interprotein interactions in collagen fibrils. Soft Matter 7:3373–3382
Pashley DH, Agee KA, Carvalho RM, Lee KW, Tay FR, Callison TE (2003) Effects of water and water-free polar solvents on the tensile properties of demineralized dentin. Dent Mater 19:347–352
Samuel J, Sinha D, Zhao JC, Wang X (2014) Water residing in small ultrastructural spaces plays a critical role in the mechanical behavior of bone. Bone 59:199–206
Unger S, Blauth M, Schmoelz W (2010) Effects of three different preservation methods on the mechanical properties of human and bovine cortical bone. Bone 47:1048–1053
Borthakur A, Reddy R, Wehrli FW (1998) NMR studies of exchangeable hydrogen in bone. In: Proceedings of the 6th annual meeting of ISMRM, Sydney, Australia, p 1804
Fernandez-Seara MA, Wehrli SL, Takahashi M, Wehrli FW (2004) Water content measured by proton-deuteron exchange NMR predicts bone mineral density and mechanical properties. J Bone Miner Res 19:289–296
Fantazzini P, Brown RJ, Borgia GC (2003) Bone tissue and porous media: common features and differences studied by NMR relaxation. Magn Reson Imaging 21:227–234
Wang XD, Ni QW (2003) Determination of cortical bone porosity and pore size distribution using a low field pulsed NMR approach. J Orthop Res 21:312–319
Ni QW, King JD, Wang XD (2004) The characterization of human compact bone structure changes by low-field nuclear magnetic resonance. Meas Sci Technol 15:58–66
Ni QW, Nyman JS, Wang XD, De Los Santos A, Nicolella DP (2007) Assessment of water distribution changes in human cortical bone by nuclear magnetic resonance. Meas Sci Technol 18:715–723
Nyman JS, Ni Q, Nicolella DP, Wang X (2008) Measurements of mobile and bound water by nuclear magnetic resonance correlate with mechanical properties of bone. Bone 42:193–199
Horch RA, Nyman JS, Gochberg DF, Dortch RD, Does MD (2010) Characterization of 1H NMR signal in human cortical bone for magnetic resonance imaging. Magn Reson Med 64:680–687
Fernandez-Sara MA, Wehrli SL, Wehrli FW (2002) Diffusion of exchangeable water in cortical bone studied by nuclear magnetic resonance. Biophys J 82:522–529
Ong HH, Wright AC, Wehrli FW (2012) Deuterium nuclear magnetic resonance unambiguously quantifies pore and collagen-bound water in cortical bone. J Bone Miner Res 27:2573–2581
Gatehouse PD, Bydder GM (2003) Magnetic resonance imaging of short T2 components in tissue. Clin Radiol 58:1–19
Reichert IL, Robson MD, Gatehouse PD, He T, Chappell KE, Holmes J, Girgis S, Bydder GM (2005) Magnetic resonance imaging of cortical bone with ultrashort TE pulse sequences. Magn Reson Imaging 23:611–618
Techawiboonwong A, Song HK, Leonard MB, Wehrli FW (2008) Cortical bone water: in vivo quantification with ultrashort echo-time MR imaging. Radiology 248:824–833
Du J, Diaz E, Carl M, Bae W, Chung CB, Bydder GM (2012) Ultrashort echo time imaging with bicomponent analysis. Magn Reson Med 67:645–649
Du J, Hamilton G, Takahashi A, Bydder M, Chung CB (2007) Ultrashort echo time spectroscopic imaging (UTESI) of cortical bone. Magn Reson Med 58:1001–1009
Li C, Seifert AC, Rad HS, Bhagat YA, Rajapakse CS, Sun W, Lam SC, Wehrli FW (2014) Cortical bone water concentration: dependence of MR imaging measures on age and pore volume fraction. Radiology 272:796–806
Horch RA, Gochberg DF, Nyman JS, Does MD (2012) Clinically compatible MRI strategies for discriminating bound and pore water in cortical bone. Magn Reson Med 68:1774–1784
Manhard MK, Horch RA, Gochberg DF, Nyman JS, Does MD (2014) Reproducibility of in vivo bound and pore water imaging of cortical bone. In: ISMRM Annual Meeting, Milan
Manhard MK, Horch RA, Harkins KD, Gochberg DF, Nyman JS, Does MD (2014) Validation of quantitative bound- and pore-water imaging in cortical bone. Magn Reson Med 71:2166–2171
Burghardt AJ, Kazakia GJ, Ramachandran S, Link TM, Majumdar S (2010) Age- and gender-related differences in the geometric properties and biomechanical significance of intracortical porosity in the distal radius and tibia. J Bone Miner Res 25:983–993
Bousson V, Meunier A, Bergot C, Vicaut E, Rocha MA, Morais MH, Laval-Jeantet AM, Laredo JD (2001) Distribution of intracortical porosity in human midfemoral cortex by age and gender. J Bone Miner Res 16:1308–1317
Horch RA, Gochberg DF, Nyman JS, Does MD (2011) Non-invasive predictors of human cortical bone mechanical properties: T2-discriminated 1H NMR compared with high resolution X-ray. PLoS ONE 6:e16359
Granke M, Makowski AJ, Uppuganti S, Does MD, Nyman JS (2015) Identifying novel clinical surrogates to assess human bone fracture toughness. J Bone Miner Res. doi:10.1002/jbmr.2452
Granke M, Grimal Q, Saied A, Nauleau P, Peyrin F, Laugier P (2011) Change in porosity is the major determinant of the variation of cortical bone elasticity at the millimeter scale in aged women. Bone 49:1020–1026
Bae WC, Chen PC, Chung CB, Masuda K, D’Lima D, Du J (2012) Quantitative ultrashort echo time (UTE) MRI of human cortical bone: correlation with porosity and biomechanical properties. J Bone Miner Res 27:848–857
Rai RK, Barbhuyan T, Singh C, Mittal M, Khan MP, Sinha N, Chattopadhyay N (2013) Total water, phosphorus relaxation and inter-atomic organic to inorganic interface are new determinants of trabecular bone integrity. PLoS ONE 8:e83478
Wehrli FW (2013) Magnetic resonance of calcified tissues. J Magn Reson 229:35–48
Delmas PD, Genant HK, Crans GG, Stock JL, Wong M, Siris E, Adachi JD (2003) Severity of prevalent vertebral fractures and the risk of subsequent vertebral and nonvertebral fractures: results from the MORE trial. Bone 33:522–532
Brennan TC, Rizzoli R, Ammann P (2009) Selective modification of bone quality by PTH, pamidronate, or raloxifene. J Bone Miner Res 24:800–808
Tasci A, Bilgili H, Altunay H, Gecit MR, Keskin D (2010) Biomechanical and histological outcome of combined raloxifene-estrogen therapy on skeletal and reproductive tissues. Eur J Pharmacol 627:354–361
Allen MR, Iwata K, Sato M, Burr DB (2006) Raloxifene enhances vertebral mechanical properties independent of bone density. Bone 39:1130–1135
Allen MR, Hogan HA, Hobbs WA, Koivuniemi AS, Koivuniemi MC, Burr DB (2007) Raloxifene enhances material-level mechanical properties of femoral cortical and trabecular bone. Endocrinology 148:3908–3913
Gallant MA, Brown DM, Hammond M, Wallace JM, Du J, Deymier-Black AC, Almer JD, Stock SR, Allen MR, Burr DB (2014) Bone cell-independent benefits of raloxifene on the skeleton: a novel mechanism for improving bone material properties. Bone 61:191–200
Anumula S, Wehrli SL, Magland J, Wright AC, Wehrli FW (2010) Ultra-short echo-time MRI detects changes in bone mineralization and water content in OVX rat bone in response to alendronate treatment. Bone 46:1391–1399
Bae WC, Patil S, Biswas R, Li S, Chang EY, Statum S, D’Lima DD, Chung CB, Du J (2014) Magnetic resonance imaging assessed cortical porosity is highly correlated with muCT porosity. Bone 66:56–61
McCalden RW, McGeough JA, Barker MB, Court-Brown CM (1993) Age-related changes in the tensile properties of cortical bone. The relative importance of changes in porosity, mineralization, and microstructure. J Bone Joint Surg Am 75:1193–1205
Yeni YN, Brown CU, Wang Z, Norman TL (1997) The influence of bone morphology on fracture toughness of the human femur and tibia. Bone 21:453–459
Currey JD, Brear K, Zioupos P (1996) The effects of ageing and changes in mineral content in degrading the toughness of human femora. J Biomech 29:257–260
Keller TS, Mao Z, Spengler DM (1990) Young’s modulus, bending strength, and tissue physical properties of human compact bone. J Orthop Res 8:592–603
McNerny EM, Gong B, Morris MD, Kohn DH (2014) Bone fracture toughness and strength correlate with collagen cross-link maturity in a dose-controlled lathyrism mouse model. J Bone Miner Res 30:446–455
Vashishth D, Gibson GJ, Khoury JI, Schaffler MB, Kimura J, Fyhrie DP (2001) Influence of nonenzymatic glycation on biomechanical properties of cortical bone. Bone 28:195–201
Tang SY, Zeenath U, Vashishth D (2007) Effects of non-enzymatic glycation on cancellous bone fragility. Bone 40:1144–1151
Kopp J, Bonnet M, Renou JP (1990) Effect of collagen crosslinking on collagen-water interactions (a Dsc investigation). Matrix 9:443–450
Miles CA, Avery NC, Rodin VV, Bailey AJ (2005) The increase in denaturation temperature following cross-linking of collagen is caused by dehydration of the fibres. J Mol Biol 346:551–556
Acknowledgements and Funding
Our work investigating the role of water in bone is made possible by funding from The National Institute of Arthritis and Musculoskeletal and Skin Diseases and The National Institute of Biomedical Imaging and Bioengineering of the National Institutes of Health (NIH) under award nos. AR063157 and EB014308, respectively. Additional support is provided by a Biomedical Laboratory Research and Development grant 1I01BX001018 from the VA Office of Research and Development. We thank the Musculoskeletal Tissue Foundation (Edison, NJ) and the Vanderbilt Donor Program (Nashville, TN) for providing cadaveric bone. We thank Mary Kate Manhard for providing the images of bound water and pore water from the tibia of a subject.
Conflict of interest
Dr. Nyman and Dr. Does have a patent entitled "System and Method for Determining Mechanical Properties of Bone Structures". This patent involves measurements of bound and pore water. Dr. Granke’s salary is supported by AR063157, but otherwise has no conflicts related to the present research.
Human and Animal Rights and Informed Consent
The imaging of subjects followed a protocol approved by the Institutional Review Board at Vanderbilt University. The use of animals followed a protocol approved by the Institutional Animal Care and Use Committee at Vanderbilt University.
Author information
Authors and Affiliations
Corresponding author
Rights and permissions
About this article
Cite this article
Granke, M., Does, M.D. & Nyman, J.S. The Role of Water Compartments in the Material Properties of Cortical Bone. Calcif Tissue Int 97, 292–307 (2015). https://doi.org/10.1007/s00223-015-9977-5
Received:
Accepted:
Published:
Issue Date:
DOI: https://doi.org/10.1007/s00223-015-9977-5